Welcome to the latest installment of Pontem’s core concepts within traditional flow assurance challenges. This week, we turn our attention to a major component of crude oil, which has numerous uses and applications, yet can be problematic in production scenarios: asphaltenes. My last post was focused on another major component of crude oil, namely wax, which you can find in the link below.
Introduction to Asphaltenes
Asphaltenes are characterized as a solubility class of compounds, specifically insoluble in aliphatic solvents (such as heptane or pentane) and soluble in aromatic solvents (such as xylene or benzene). They do not have a defined composition nor a defined structure, although they have a few common traits, which include: high molecular weight, polar, aromatic compounds often containing heterocyclic components and aliphatic chains throughout the cyclic morphology.
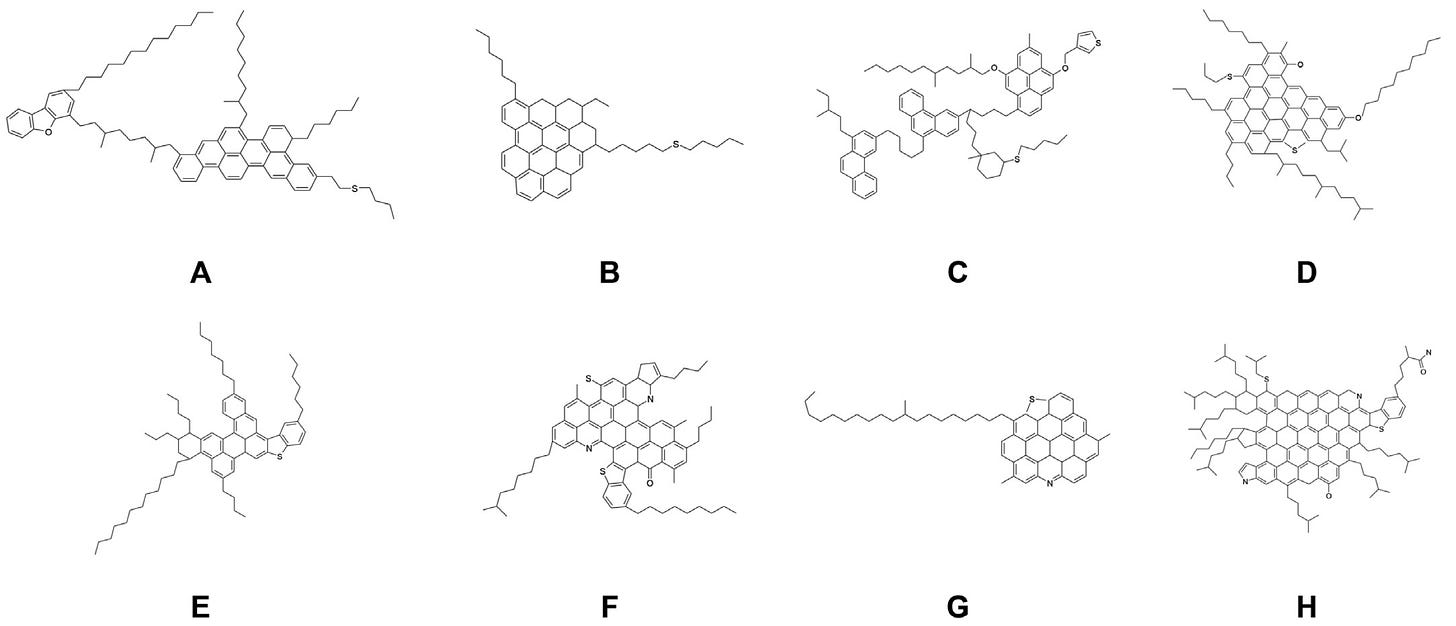
Asphaltenes are stabilized in crude oil through associations with resins and aromatic components in the live fluid as it exists under reservoir conditions. Absent of those interactions asphaltenes would self-associate into massive aggregates. However, under reservoir conditions asphaltenes don’t self-associate as they are kept in a lower energy state through those interactions with the other fluid components. After all, the thermodynamic stability of the fluid has been established over a geologic timescale!
And Then Everything Changed…
Once a reservoir is drilled, completed, and production begins, the thermodynamic stability of the fluid shifts as it is exposed to the changing pressures and temperatures along the production train (from the reservoir up the wellbore, through the flowline, into the fluids processing vessels, and eventually to downstream processing facilities). The physical environment that created a temperature and pressure equilibrium for asphaltenes will shift, and sometimes that can lead to instability, precipitation, and even deposition. This isn’t as simple as the oil being either above or below the melting temperature as in the case of wax. The destabilization of asphaltenes is a more complicated process.
Let’s start with an overview of what happens to the single phase fluid as it leaves the reservoir, and what those changes mean for asphaltene stability. First, temperatures and pressures will begin to decrease, and as that occurs the fluid begins to expand. At this point, it is still a single-phase fluid and the composition of the fluid is the same as it was in the reservoir. However, impact of the expanded volume results in a portion of the fluid gaining molar volume by a significant percentage of the entire fluid. Here, I’m talking about the short carbon chain molecules (methane, ethane, propane, etc.) as well as carbon dioxide and nitrogen. That increase in molar volume fraction begins to destabilize asphaltenes. Recall that I mentioned asphaltenes are insoluble in aliphatic solvents. Well, those short hydrocarbons are acting in the same way. Asphaltenes will begin to form aggregates and precipitate out of solution, and the point at which that happens is commonly referred to as the Asphaltene Onset Pressure (AOP). One can literally map the pressure gradient along the wellbore and by doing so determine the exact depth where asphaltenes will begin to precipitate.
Asphaltenes will continue to destabilize as the fluids cross the bubble-point, which is the pressure/temperature at which gas breaks out and the fluid becomes multi-phase with defined gas and liquid phases (and a solid phase, technically, if asphaltenes are dropping out!). As pressures and temperatures continue to decrease, light hydrocarbons will continue to break out of the liquid hydrocarbon phase, prompting an interesting phenomena to occur: the molar volume of aromatics and resins will increase in the liquid phase, which eventually helps to stabilize the asphaltenes again. Keep in mind, the timescale is too short to allow for asphaltenes to “re-dissolve” but they will stop precipitating. In other words, it is often the case that not all asphaltenes will precipitate as the fluids cross the asphaltene onset pressure. The amount of asphaltenes that flocculate out of the crude oil and the conditions that facilitate asphaltene instability vary widely from system to system. There are systems with high percentages of asphaltenes with no reported issues, and there are crude oils with small amounts of asphaltenes that require severe mitigation and remediation programs. This shifting stability characteristic even muddies the reported asphaltene content in some oil characterization reports: the asphaltene content changes if pentane (C5) is used vs. heptane (C7) to isolate asphaltenes.
The introduction presented above begs a couple of questions: first, what are mitigation options to prevent asphaltene deposition? And, second, how is the AOP measured? Let’s start with the AOP.
Determining the AOP
The value of understanding when and where asphaltenes will precipitate is significant when considering field development, in general, and specifically mitigation programs to reduce/eliminate asphaltene fouling risks. Consider new field developments for a moment: knowing the AOP location will allow one to install a chemical injection point upstream of where asphaltenes are predicted to foul, which will help design the most effective asphaltene treatment plan possible. So, how does one go about measuring a fluid’s AOP?
If during exploration stages enough forethought and funding allows for collecting a live oil sample and running tests to measure the AOP, then there are several options available. A brief summary of a few live-oil tests are listed below.
Gravimetric Method via Isothermal Depressurization
A single-phase reservoir sample is equilibrated to a temperature in a PVT cell. It is important the pressure of the cell is above the reservoir temperature so as to avoid prematurely precipitating asphaltenes. In multiple step-wise depressurization and equilibration steps the fluid is exposed to lower pressures. The experiment is set to allow any destabilized asphaltenes to agglomerate and settle to the bottom of the cell. Small samples are pulled prior to agitating and lowering the pressure further. The asphaltene content of the aliquots are measured. The concentration of asphaltenes will decrease in the aliquot once the fluids pass below the AOP. This technique is accurate and useful for determining the upper and lower asphaltene phase boundaries, as well as the fluid bubble point. As one can imagine, however, the technique is time consuming, expensive, and can consume a fair volume of sample.
Organic Solids Deposition and Control Device (OSDCD)
Also known as RealView, this instrument measures the rate of deposition of waxes and asphaltenes from live oil samples through various shear rate and temperature conditions. Two concentric cylinders are employed where the inner cylinder rotates at high speeds to force fluid flow, while the stationary, outer cylinder acts as the flowline wall. The continuous flow of fresh oil allows for deposits to be collected against the outer wall as depressurization occurs.
Near Infrared Light Scattering Measurement (NIR-LSM)
This technique incorporates a laser focused through a visual cell containing the pressurized fluids. In order to maximize the utility of this technique, particularly with dark colored oils, NIR light ranging 800-2200 nm wavelength light is employed. The PVT cell is either depressurized or the volume of the cell is expanded (Constant Mass Expansion test), which also serves to destabilize asphaltenes. Similar to the gravimetric method, each change in pressure (or volume) is coupled with an equilibration period. As opposed to the gravimetric method, NIR is looking for the formation of aggregates rather than the depletion of asphaltenes from the single phase liquid. The aggregation and precipitation of asphaltenes is detected through light scattering detection via fiber optics. The detection of asphaltene onset can be subjective, depending on the system, however advantages such as speed of experimentation and sample size requirements makes this technique a favorable one.
Organic Deposition Cell (ODC)
The ODC utilizes many of the same principles as the NIR set-up, except the light source is different. The asphaltene flocculation is measured via fiber optics in which a luminescent spectrophotometer detects the light scattering. This technique is not as widely-used as the NIR detection systems due to the fact the NIR light source is more penetrating through the live oil sample.
Laboratory Summary for Live-Oil AOP Measurements
This list is by no means exhaustive and only meant to provide a high-level summary of tools available if single phase reservoir fluids are available. The obvious limitation of all techniques for AOP measurement is the results say nothing to the possibility of deposition. The results only describe the conditions by which asphaltenes will begin to destabilize and precipitate into particles.
The exercise of collecting reservoir fluids can be costly and disruptive to operations if the field is producing. So, if there aren’t any live oil samples available what are the options for measuring the AOP?! …This lead in comes with a spicy take: Joe Cocker’s cover of The Beatle’s, “With a Little Help From My Friends” is better than the original.
STO Samples for AOP Measurements - ASIST
Did you see what I did there?! Anywho, what can be done if there aren’t live oil samples available for AOP measurements? The ASIST (ASphaltene InStability Trend) methodology was developed by Prof. Jill Buckley at New Mexico Tech and Chevron in 1998, which established the asphaltene onset conditions change predictably with the molar volume of n-alkane solvents. Within the methodology, the asphaltene onset is defined by the refractive index (RI) of the mixture (oil, flocculant, and precipitated asphaltene). It is important to note the refractive index of the mixture can be thought of as a defined physical property, which then allows one to extrapolate the RI trends to reservoir conditions in order to predict the asphaltene destabilization events during depressurization.
n-Alkanes to destabilize asphaltenes
So, there’s a lot to unpack here! Let’s start with what happens when adding n-alkane solvents to crude oil. Through the 1990’s and early 2000’s, quick bench top tests, known as ADT’s (asphaltene dispersant tests), were used as first approximations to determine whether a system would have asphaltene challenges. The test essentially mixed 100-300 ul crude into 10 ml heptane (note the volumes!) and over time observations were made to see if asphaltenes precipitated.
The ASIST model took a more refined approach in utilizing the Flory-Huggins thermodynamic model describing asphaltene molecules in small solvents. By titrating a crude oil at a fixed temperature over defined timelines, an asphaltene flocculation point could be measured. At the exact moment of destabilization, the refractive index is recorded, which is used to characterize that mixture. I’ve described this as “the exact moment” as opposed to “onset” to emphasize the care one needs to take in the laboratory. The total test matrix is general run with multiple solvents (C7, C11, and C15), at multiple temperatures (40°C and 70°C) and multiple timepoints (20 min, 5 hours, and 24 hours). Taken together, the full kinetic and thermodynamic stability of the asphaltenes in question becomes clear. With those known ratios in hand, molar volume estimates can be made for a regression analysis.
Fluid Characterization Under Pressure
ASIST involves a series of flash calculations of the fluids using data reported in a standard PVT report, as well as flash calculations of the solvents used in the laboratory. Specifically, the flash calculations will provide molar volume calculations under various pressure/temperature conditions, including STP conditions. And a regression analysis is run on the live crude oil to correlate the molar volume of fluids during depressurization to a predicted RI value at pressures during depressurization, which is based on a measured RI of the crude oil at STO conditions.
Similarly, flash calculations of the solvents used during the titrations can be combined with the crude oil molar volume calculations to calculate a predicted RI value under pressure at each time point, which yields kinetic profiles of the asphaltene stability as a function of pressure.
Bringing It Together
Plotting the live oil RI and the predicted RI based on the titrations (PRI) as a function of pressure will generate a plot similar to that shown below. When the Live Oil RI (orange dataset below) is above the PRI dataset (purple), it indicates asphaltenes are stable in solution. When Live Oil RI drops below the PRI value, it indicates asphaltenes are unstable and will precipitate out of solution. Recall, as a fluid is produced, that is leaving the reservoir, it will depressurize, so the chart should be interpreted by reading the Live Oil RI dataset from right to left. The AOP is then the point at which the Live Oil RI crosses the PRI line. As mentioned earlier, after passing through the bubble point, the molar volume of destabilizing components are no longer in the same phase as the asphaltenes and therefore, asphaltenes are no longer destabilized. This is noted with the Lower AOP point, where the Live Oil RI crosses the PRI again. For simplicity, the chart example below only shows as single PRI timepoint. In reality, each kinetic point (20 min, 5 hr, 24 hr) will yield a PRI dataset, which essentially will provide up to 3 upper AOP values. The reported AOP value will therefore be a range, which represents a thermodynamic picture of the asphaltene stability in the system.
How Does Titrations Correlate With Live Oil Depressurization?
While it may be intuitive to measure physical properties of mixtures at STP conditions and predict the value at high pressures/temperatures, is it valid to compare asphaltene instability observed during depressurization and asphaltene instability from the addition of n-alkane solvents at ambient pressure? There were a number of published results demonstrating ASIST predictions to be in good agreement with field results, however they might have been viewed as ideal cases with good correlations.
In 2003, Ting utilized a model crude oil (1% isolated asphaltenes in toluene) reconstituted with increasing methane content gas to measure asphaltene onset pressures as a function of methane content via light scattering techniques (Ting, P.D. 2003. “Thermodynamic stability and phase behavior of asphaltenes in oil and of other highly asymmetric mixtures,” PhD thesis, Rice University, Houston, Texas).
Interestingly, Buckley, et al. found similar AOP predicted values of the model system using ASIST (Buckley, J.S., Wang, J., and Creek, J.L. 2007. “Solubility of the least soluble asphaltenes.” In Asphaltenes, Heavy Oils and Petroleomics, ed. O.C. Mullins, E.Y. Sheu, A. Hammami, and A.G. Marshall, Chap. 16, 401–437. New York: Springer Science+Business Media.), which was the first demonstration n-alkanes could be used to simulate asphaltene destabilization phenomena during depressurization.
AOP Advantages And Wrapping Up
One disadvantage live oil measurements have is the results are valid for the exact fluid measured. With asphaltene stability, there are a number of changes in the field that limit the applicability of those results. For example, later in life fields will also require gas lift operations, which will change the molar volume of destabilizing components. Or production systems will open up different zones to the production train, which may impact asphaltene stability.
ASIST offers increased utility because the results are based on molar volume calculations and regression analyses. What that means is a gas lift stream or another crude oil stream can be added in the post-experimental work-up (no additional lab tests!) and the impact can be evaluated. The downside is the results provide a range of values that may be interpreted as too broad. While technically correct, having a grey answer rather than an exact number may not feel great.
At Pontem, we remain agnostic about the tools we employ to make sense of data and drive impactful decision making. We’ve gone over several options that provide an AOP. So, now what?! Tune in next time and we’ll discuss how to manage asphaltenes (chemically and mechanically), what happens when production streams are commingled or gas lifts are added, which are destabilizing for asphaltenes.